3. Mechanisms of DNA repair and synthesis
Gene Machine: DNA polymerase
DNA polymerases are dynamic molecular machines that faithfully copy DNA during DNA replication and repair.[1] One of the important properties of this enzyme is its remarkable fidelity, which is due to a series of non-covalent transitions that precede the chemical step of phosphoryl transfer and serve as kinetic checkpoints, rejecting inappropriate substrates early in the reaction pathway[2]. An important conformational change is the “fingers-closing” transition, inferred from cocrystal structures, in which the addition of the correct complementary deoxyribonucleotide (dNTP) to a polymerase-DNA (Pol-DNA) binary complex results in a transition from an open to a closed conformation, forming a snug binding pocket around the nascent base pair. The small magnitude of such conformational changes, coupled with the transient nature of the intermediates, makes ensemble study the fingers-closing transitions especially difficult.
To get a dynamic view of the DNA polymerase machinery and its conformational changes, we use single-molecule FRET (smFRET) techniques, including alternating laser excitation (ALEX) and Fluorescence Correlation Spectroscopy (FCS), both in solution as well as in gels [3, 4]. Our first single-molecule FRET studies of E. coli DNA polymerase I by Santoso et al.[3] captured conformational transitions crucial for polymerase accuracy. Using DNA polymerase I (Klenow fragment) labelled with both donor and acceptor fluorophores, we studied conformational transitions that precede nucleotide addition. Our experiments clearly distinguished the open and closed conformations that predominate in crystal structures of Pol-DNA and Pol-DNA-dNTP complexes, respectively. By contrast, the unliganded polymerase showed a broad distribution of FRET values, indicating a surprisingly high conformational flexibility in the protein in the absence of its substrates. Ternary complexes formed in the presence of mismatched dNTPs or complementary ribonucleotides showed novel FRET species, which we suggested are relevant to kinetic checkpoints that discriminate against these incorrect substrates. This original studied was confirmed by further structural and biophysical studies.
We used the same approach to study various fidelity mutants and highlight mechanisms by which active-site side chains influence the conformational transitions and free-energy landscape that underlie fidelity decisions in DNA synthesis [5]. We showed that ternary complexes of high fidelity derivatives with complementary dNTPs adopt mainly a fully closed conformation, whereas a conformation with a FRET value between those of open and closed is sparsely populated. This intermediate-FRET state, which we attributed to a partially closed conformation, was also predominant in ternary complexes with incorrect nucleotides and, strikingly, in most ternary complexes of low-fidelity derivatives for both correct and incorrect nucleotides. The mutator phenotype of the low-fidelity derivatives correlated well with reduced affinity for complementary dNTPs and highlights the partially closed conformation as a primary checkpoint for nucleotide selection.
We also extended our work to surface-immobilized complexes to observe fingers-opening and closing of individual polymerase molecules in real time [6]. Our results revealed the presence of intrinsic dynamics in the binary complex, characterized by slow fingers-closing and fast fingers-opening. When binary complexes were incubated with increasing concentrations of complementary nucleotide, the fingers-closing rate increased, strongly supporting an induced-fit model for nucleotide recognition. Meanwhile, the opening rate in ternary complexes with complementary nucleotide was 6 s−1, much slower than either fingers closing or the rate-limiting step in the forward direction; this rate balance ensured that, after nucleotide binding and fingers-closing, nucleotide incorporation is overwhelmingly likely to occur. Our results for ternary complexes with a non-complementary dNTP confirmed the presence of a state corresponding to partially closed fingers and suggested a radically different rate balance regarding fingers transitions, which allows polymerase to achieve high fidelity.
In work being prepared for publication (Craggs et al), we also recovered the 3D structure and conformational dynamics of a DNA polymerase complex with a gapped DNA-repair substrate; this work was done using systematic FRET measurements and molecular dynamics simulations. This work provides insight on how structure-specific proteins such as DNA polymerase identify their DNA targets.
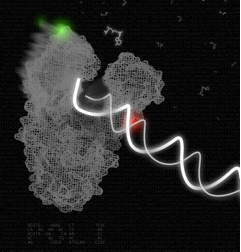
An artistic rendition of the DNA polymerase in action. In this picture, the polymerase-DNA binary complex undergoes the ‘fingers-closing’ transition in the presence of incoming complementary nucleotide. The polymerase-DNA structure shown is based on the B. stearothermophilus DNA polymerase (PDB file 1L3U); the green and red spheres show the positions of the fluorophores. I
- C.M. Joyce. Encyclopedia of Biological Chemistry, chapter DNA polymerase I, bacterial, pages 720–725. Elsevier, 2004.
- C.M. Joyce and S.J. Benkovic. DNA polymerase fidelity: kinetics, structure, and checkpoints. Biochemistry, 43(45):14317–14324, Nov 2004.
- Y. Santoso, C.M. Joyce, O. Potapova, L. Le Reste, J. Hohlbein, J.P. Torella, N.D.F. Grindley, and A.N. Kapanidis. Conformational transitions in DNA polymerase I revealed by single-molecule FRET. Proc. Natl. Acad. Sci. U. S. A., 107(2):715–720, 2010.
- Y. Santoso and A.N. Kapanidis. Probing Biomolecular Structures and Dynamics of Single Molecules Using In-Gel Alternating-Laser Excitation. Anal. Chem., 81(23):9561–9570, 2009.
- Hohlbein J, Aigrain L, Craggs TD, Bermek O, Potapova O, Shoolizadeh P, Grindley NDF, Joyce CM, and Kapanidis AN, Conformational landscapes of DNA polymerase I and mutator derivatives establish fidelity checkpoints for nucleotide insertion, Nature Communications, 2013; 4, 2131.
- Evans GW, Hohlbein J, Craggs T, Aigrain L, Kapanidis AN. Real-time single-molecule studies of the motions of DNA polymerase fingers illuminate DNA synthesis mechanisms. Nucleic Acids Research, 2015. gkv547