1. DNA Biosensors
Robert Crawford, Achillefs Kapanidis
DNA is an extremely versatile material with which to build biosensors; molecules can be encoded with binding sites specific for proteins of interest, or for other nucleic acids (DNA/RNA), and can be easily modified to contain useful chemical groups such as fluorophores, biotin or quantum dots. Employing self-assembly properties of DNA, one can also design topological features to create different 2- or 3-dimensional shapes of sizes similar to biomolecular targets. This additional layer of freedom allows even more creativity when designing biosensors.
Our lab develops biosensors for the detection of DNA-binding proteins and nucleic acids.
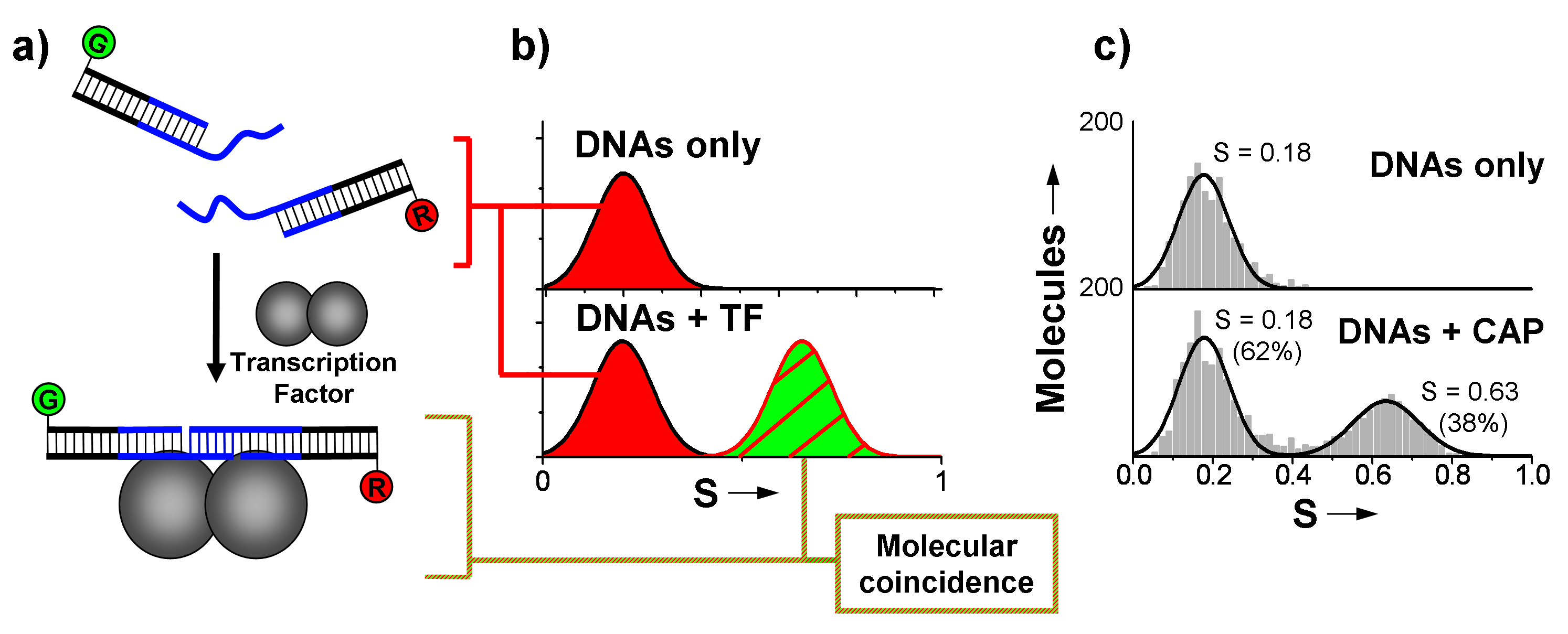
Figure 1. Half-site based transcription factor (TF) detection. Association of the two DNA fragments in the presence of the specific TF yields an observable change in stoichiometry using ALEX spectroscopy.
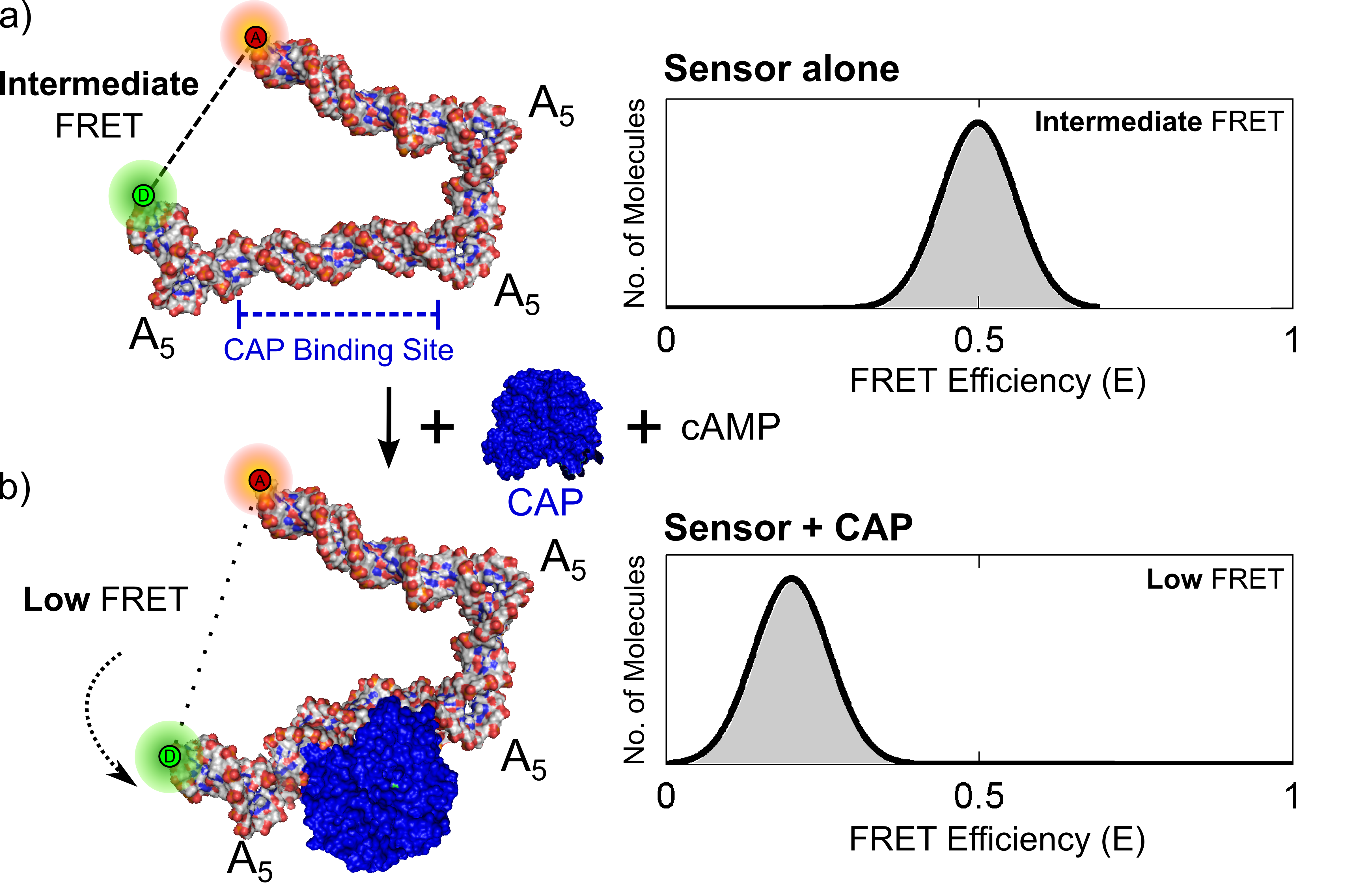
Figure 2. FRET-based transcription factor detection (TF). TFs known to bend DNA such as catabolite activator protein (CAP) can be detected using such a design. Three A5 kinks in the DNA bring the ends into a detectible FRET range. TF presence is indicated by bending of one edge.

Figure 3. Nucleic acid biosensing. In its simplest form can be formed from 1-2 labeled capture probes encoding the reverse complement of the target sequence.
- Lymperopoulos K*, Crawford R*, Torella JP, Heilemann M, Hwang LC, Holden SJ, and Kapanidis AN, Single-molecule DNA biosensors for protein and ligand detection. Angew. Chem. 2010; 49 (7), pp.1316-1320. *equal contribution.
- Crawford R, Kelly DJ, Kapanidis AN, A Protein Biosensor That Relies on Bending of Single DNA Molecules, ChemPhysChem 2012; 13 (4), pp.918-922.
2. DNA as a Photonic Waveguide
David Bauer, Achillefs Kapanidis
Light-harvesting complexes found in nature are vivid examples of nanoscale photonic waveguides. After an absorption of a photon by pigment-protein complexes, energy is transfered through a series of radiationless transfer to the transmembrane reaction centre complex. DNA is a good candidate material for such photonic wires, mainly due to the availability of several labelling strategies for introducing chromophores on DNA (Refs. 1-3). We are currently working on improving the generality and transfer efficiency of DNA photonic wires by using (1) homogeneous repeat elements; (2) a cascade of spectrally different fluorophores; and (3) non-specific fluorophores as energy transfer intermediates.
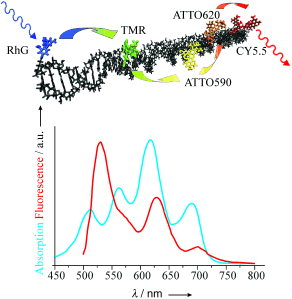
A nanometer-sized molecular photonic wire based on DNA as rigid scaffold (upper panel), along with the absorption and emission spectra of the fluorophore used (lower panel). (Tinnefeld et al., 2005)
- Heilemann M, Tinnefeld P, Mosteiro GS, Parajo MG, Van Hults NG, and Sauer M, Multistep energy transfer in single molecular photonic wires. J. Am. Chem. Soc. 2004; 126, pp.6514-6515.
- P. Tinnefeld, M. Heilemann, and M. Sauer. Design of molecular photonic wires based on multistep electronic excitation transfer. Chem. Phys. Chem. 2005; 6, pp.217-222.
- S. Vyawahare, S. Eyal, K.D. Mathews, and S.R. Quake. Nanometer-scale fluorescence resonance optical waveguides. Nano lett. 2004; 4, pp.1035-1039.
3. DNA cages for transcription factors
Robert Crawford, Achillefs Kapanidis
This project is a collaboration with The DNA group led by Prof Andrew Turberfield (also Oxford Physics Link) and extends successful work on a DNA tetrahedron [1]. In this case we harness the self-assembly properties of DNA to produce a cage that encapsulates the transcription factor catabolite activator protein (Fig 1; [1]). Such a protein encapsulating cage could be used as a drug delivery vehicle or for protein structural studies using FRET. We prove encapsulation and correct orientation of CAP within the cage using single-molecule FRET and demonstrate the effect of the cage at slowing the off-rate of CAP compared to a linear sequence.
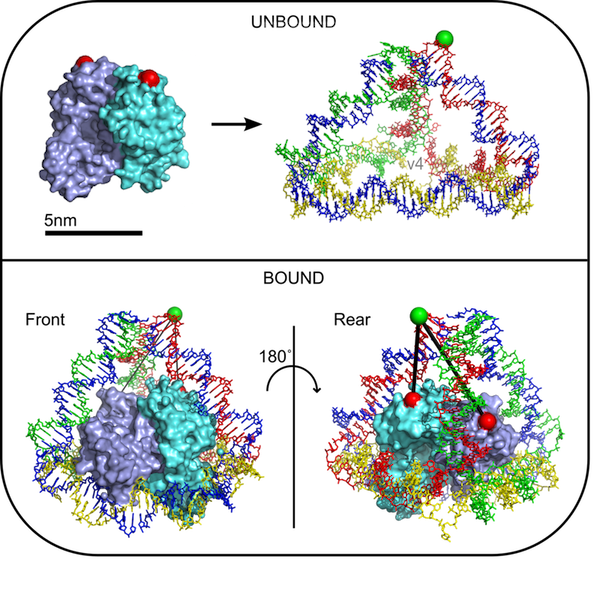
Figure 1. A nanoscale cage designed to encapsulate the transcription factor catabolite activator protein.
- Goodman RP, Heilemann M, Doose S, Erben CM, Kapanidis AN, Turberfield AJ, Reconfigurable, braced, three-dimensional DNA nanostructures. Nat Nanotechnol. 2008; 3(2), pp.93-6.
- Crawford R, Erben CM, Periz J, Hall LM, Brown T, Turberfield AJ, and Kapanidis AN, Non-covalent Single Transcription Factor Encapsulation Inside a DNA Cage. Angew. Chem. (accepted, forthcoming).